Thymine DNA Glycosylase: a key player in DNA base excision repair
Thymine DNA glycosylase (TDG) is one of the most important enzymes in the base excision repair (BER) mechanism, pivotal for correcting erroneous DNA methylation patterns that can lead to genomic instability. This enzyme targets and repairs aberrant cytosine derivatives, specifically 5-formylcytosine (5fC) and 5-carboxylcytosine (5caC), facilitating the maintenance of epigenetic marks and gene expression profiles. Understanding the operational dynamics of TDG within chromatin contexts is crucial for elucidating its role in cellular processes and disease mechanisms.
Important proteins during base excision repair (BER)
DNA Glycosylases – These enzymes recognize and remove damaged bases through cleavage of the N-glycosidic bond, creating an abasic site (AP site). Examples include:
- Uracil DNA glycosylase (UDG)
- 8-oxoguanine DNA glycosylase (OGG1)
- Thymine DNA glycosylase (TDG)
AP Endonuclease (APE1) – This enzyme cleaves the phosphodiester backbone at the 5′ end of the abasic site, creating a nick with a 3′ hydroxyl group and a 5′ deoxyribose phosphate.
DNA Polymerase – This enzyme synthesizes new DNA to fill the gap created by the removal of the damaged base. Key polymerases in BER include:
- DNA polymerase β (Pol β) in mammals
- DNA polymerase I in prokaryotes
DNA Ligase – This enzyme seals the nick in the DNA backbone after the gap is filled. In humans, DNA ligase III usually performs this function in BER, working in conjunction with XRCC1 (X-ray repair cross-complementing protein 1).
Flap Endonuclease 1 (FEN1) – In long-patch BER, which involves the replacement of several nucleotides, FEN1 removes the RNA-DNA flap created by displaced strand synthesis.
XRCC1 (X-ray Repair Cross-Complementing Protein 1) – This protein acts as a scaffold for the assembly of other BER proteins at the site of damage, increasing the efficiency of repair.
Gaps in Understanding TDG’s Mechanistic Functionality
Traditional studies on thymine DNA glycosylase (TDG) have primarily utilized ensemble biochemical assays that average out the responses from multiple molecules. While these approaches have yielded fundamental insights, they tend to obscure the subtle interactions and behaviors of individual TDG molecules navigating complex genomic landscapes. Although structural studies have successfully illuminated the binding details between TDG and DNA molecules, they lack the ability to capture the real-time dynamics of enzymatic activity. Therefore, while these methods have laid essential groundwork, they do not fully convey the real-time dynamics and diversity of molecular interactions, especially within the context of nucleosomal DNA.
Real-Time Single-Molecule Analysis
Addressing these limitations, a recent advancement from Prof. Bennett Van Houten’s lab at University of Pittsburgh has made breakthrough discoveries using the C-Trap, a single-molecule microscopy platform. This approach offers a detailed view of the enzyme’s search dynamics, binding specificity, and repair efficiency at individual protein resolution.
By fluorescently tagging TDG and its mutants, researchers were able to monitor their activity on synthetic DNA strands designed to resemble natural DNA lesions and nucleosome-containing DNA. They employed fluorescently labeled proteins directly extracted from nuclei, eliminating the need for protein purification. Additionally, they utilized the LUMICKS DNA tethering kit, which allowed them to integrate custom sequences of interest—such as those containing a DNA lesion or a nucleosome—into their experiments with the C-Trap system. This innovative approach enabled precise and convenient tracking and analysis of the proteins’ interactions with specific DNA features.
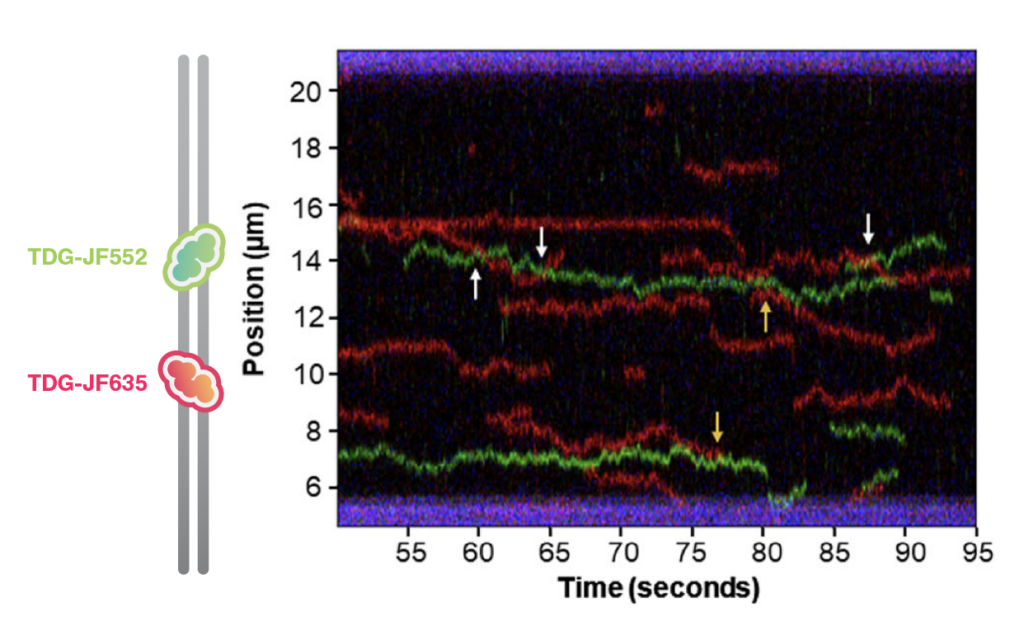
Key Discoveries: TDG’s Dual Mechanisms and Chromatin Interactions
The study’s findings elucidate several critical aspects of TDG functionality:
- Sliding and Hopping: TDG employs a combination of sliding and hopping across the DNA helix, optimizing the search efficiency along the DNA of a lesion. This bimodal searching is crucial for navigating through complex DNA environments with regulation factors or nucleosomes.
- Structural Influence: Alterations in the N-terminus and key active site residues significantly impact TDG’s binding affinity and mobility. With the direct observation of the dynamic behavior of mutants, single-molecule results were able to infer structural changes of the protein to the function, crucial for the understanding of enzymatic activity and repair actions.
- Nucleosome Dynamics: Interaction with nucleosomes varies, where TDG can either bypass, stall, or bind directly to these structural units. These activities imply that chromatin can act either as a roadblock or a scaffold during the TDG search process. Such interactions are essential for understanding how TDG accesses and repairs DNA within chromatin.
Scientific Implications and Future Directions
The insights provided by these findings significantly advance our understanding of base excision repair processes and their regulation. In this groundbreaking study, Ben van Houten’s lab revealed, for the first time, how thymine DNA glycosylase (TDG) dynamically searches for damaged DNA using sliding, hopping, and nucleosome interactions—mechanisms critical for maintaining genome stability.
The elucidation of TDG’s mechanisms invites the development of novel therapeutic strategies, particularly for diseases characterized by DNA repair deficiencies, such as cancer and age-related disorders. This research has far-reaching biological implications, answering long-standing questions about how repair enzymes navigate the complex chromatin environment—insights unattainable with traditional techniques. The C-Trap not only advances our understanding of DNA repair dynamics but also establishes a foundation for translational breakthroughs.
Images adapted from Schnable, B.L., Schaich, M.A., Roginskaya, V. et al. Thymine DNA glycosylase combines sliding, hopping, and nucleosome interactions to efficiently search for 5-formylcytosine. Nat Commun 15, 9226 (2024).