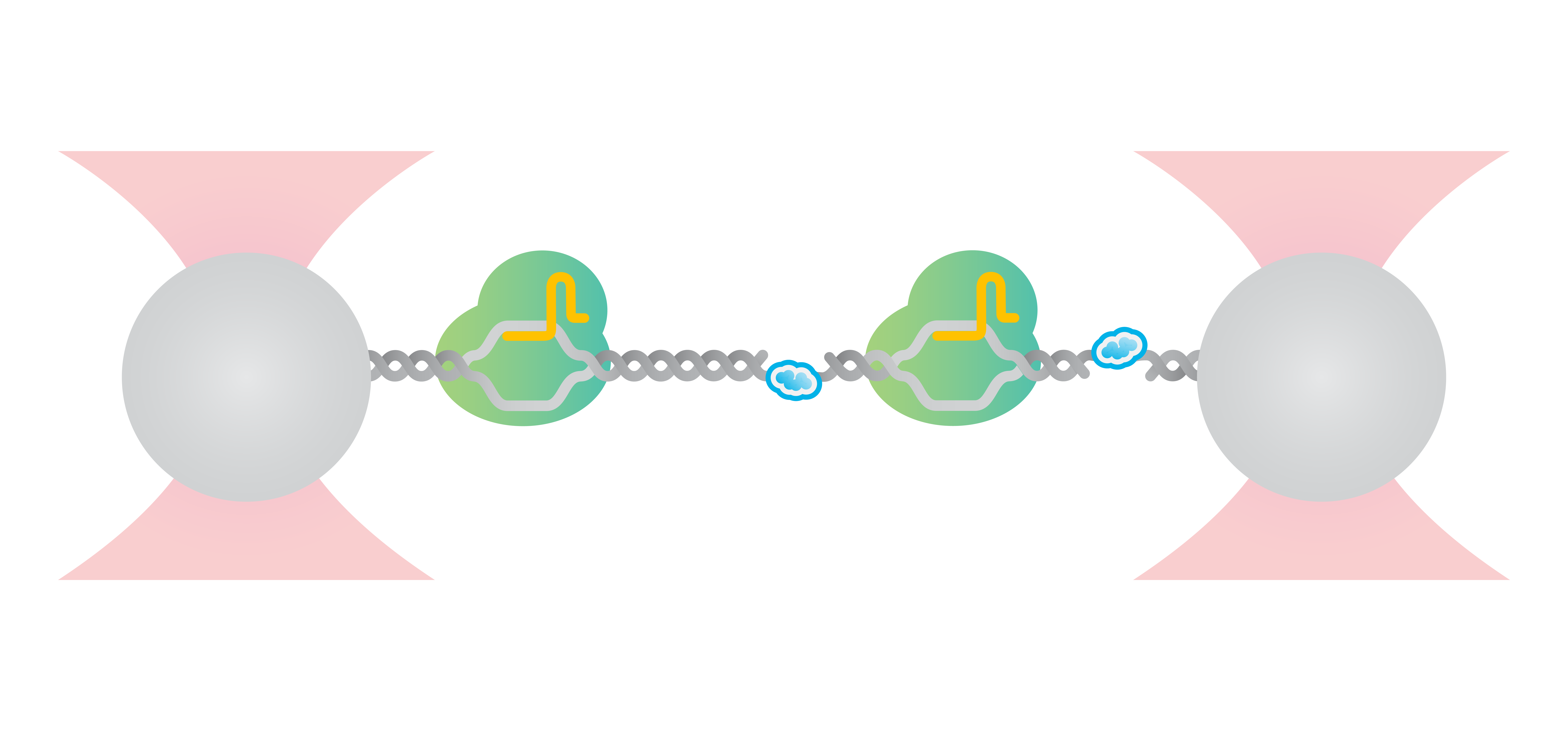
CRISPR/Cas9 is a highly popular genome-editing tool and a trending research topic. Current research is focused on fully understanding Cas9 function under different circumstances to exclude off-target binding events and ensure its precision during DNA editing applications. However, there are still many challenges in performing this research, including missing control of when and where exactly off-target events occur, together with the inability to directly visualize these binding events. For a deep understanding of the CRISPR/Cas9 mechanism and its off-target binding to DNA, it is crucial to visualize the binding location and dynamics at the base-pair level and in real-time, as well as have the tool to manipulate the DNA structure to uncover how different DNA states influence Cas9 interactions.
C-Trap® Optical Tweezers – Fluorescence & Label-free Microscopy provides exactly these capabilities, empowering your research to gather valuable insights into the detailed mechanism of CRISPR/Cas9.
Manipulation of DNA structure and real-time observation of its influence on dCas9 binding
The C-Trap is a versatile instrument that can provide a deeper understanding of the origins of Cas9 off-target binding events. In this experiment, scientists from the lab of Prof. David Rueda, used it to observe binding events while manipulating DNA structure to mimic the different DNA states that occur naturally during DNA processes in vivo. They then visualized and monitored in real-time Cas9 binding to DNA while influencing its structure, enabling direct proof of the interdependence of these interactions.
Specifically, Newton et al. used the C-Trap to tether a single double-stranded DNA (dsDNA) molecule and observe the binding of catalytically dead Cas9 (dCas9) via correlated confocal microscopy. They found that, at contour length, dCas9 bound on-target to DNA (Figure 1 top), whereas upon DNA stretching multiple off-target dCas9 binding events were observed (Figure 1 bottom).
Stretching of the DNA molecule induced the formation of “bubbles”– local openings of the DNA helix in which the dsDNA melts to single-stranded DNA (ssDNA). DNA bubbles are formed naturally during cellular processes, such as DNA replication or transcription. The results here suggest that Cas9 off-target activity is facilitated when duplex DNA is destabilized during cellular processes. The unique feature of the C-Trap to manipulate the structure of DNA by stretching the DNA molecule, as well as the real-time fluorescence measurements, allowed direct visualization and detailed investigation of the effects of DNA structure changes on dCas9 binding.
Application note | Gene editing
1 Fluorescence images showing dCas9 on-target binding to DNA at contour length (top) and additional off-target binding (bottom) to stretched DNA that has formed bubbles after applying 20 pN force. Left and right fluorescent spheres are optically trapped polystyrene beads that are holding the DNA molecule (unlabelled) in between.
Determination of dCas9 off-target selectivity within a competition DNA binding experiment
Employing the multichannel microfluidics system that is incorporated in the C-Trap, it is very easy to build an assay with multiple components by controlling its stepwise assembly. Using this capability, researchers from the lab of Prof. David Rueda isolated a DNA molecule, added human replication protein A (hRPA) and dCas9 into the flow chamber, and progressively stretched the DNA to a tension of 5 – 65 pN, creating large bubbles and DNA nicks. In this way, they created a competition experiment between dCas9 and hRPA to test dCas9 binding selectivity towards the DNA template.
The hRPA is a protein that exclusively binds to single-stranded DNA. Thus, naturally, it bound only to to the single-stranded regions (Figure 2, blue), that were created at increased stretching forces. On the contrary, dCas9 off-target binding events occurred more at intermediate stretching forces (Figure 2, green) when small DNA bubbles were present. Interestingly, the binding of both DNA-binding proteins was mutually exclusive under all conditions. These results suggest that Cas9 off-target binding is driven by small disruptions in DNA helical structure and requires the unwound DNA strands to remain in close proximity. In vivo, this occurs during processes like transient bubble melting, DNA breathing, or supercoiling.
Employing the C-Trap microfluidics system, optical tweezers and multicolor confocal microscopy enabled to make these important observations.
Application note | Gene editing
DNA-Cas9 binding experiments with genomic positions mapping in real-time
With its automated scripts for experimental runs and data analysis, the C-Trap allows a fast workflow and advanced data analysis. Furthermore, the high spatial sensitivity of the instrument allows for precise localization of DNA-binding proteins. For example, in only a few steps, it is possible to perform an experiment with multiple DNA-binding proteins and determine their binding locations at the base-pair level.
Here, we held an optically trapped DNA molecule at contour length, while observing in real-time the binding of two fluorescently labelled dCas9 proteins, green and red. We then plotted over time the binding locations of both dCas9 proteins on the DNA in a kymograph and analyzed the events using our kymotracker tool (Figure 3). This allowed us to easily map the binding events to the DNA sequence and reveal their exact genomic positions (Figure 4), by using the automated scripts for data analysis shared at harbor.

3 Kymograph displaying DNA-Cas9 interactions over time of two fluorescently labelled Cas9 proteins, one labeled with eGFP and the second one with SYTOX. The kymotracker script, available here, is applied to automatically detect the traces of the proteins (indicated on this plot by the thick green and red lines) and quantify DNA-binding events during an experiment. (LUMICKS research data)
Visit Harbor, the interactive community that colaborated in scripting and sample preparation for dynamic single-molecule experiments, and accelerate your workflow
Newton et al. (2019) Nat Struct Mol Biol
Measuring positions and strengths of dCas9-DNA interactions
Investigating DNA-Cas9 binding interactions is also possible without the need for fluorescence. Using a single-trap you can analyze the position and the strength of Cas9 binding to DNA by setting up a simple surface assay. In this measurement, a three-way DNA molecule was tethered between the substrate surface and an optically-trapped bead with a dCas9 complex bound to the trunk of the DNA. Next, the researchers from Bo Sun lab assessed the binding strength and position of the complex by unzipping the DNA through moving the coverslip away, using forward and reverse unzipping assays.
The results showed that the DNA-dCas9 connection got disrupted at well-defined locations and with distinct forces, providing information about the binding positions and strength of the interactions (Figure 5). By employing the m-Trap® optical tweezers, the scientists were able to extract information on Cas9-DNA binding strengths, determine the base pair location of the binding, and investigate the influence of additional DNA-binding complexes from a single experiment.
5 Representative force traces of forward (left) and reverse (right) DNA unzipping in presence of dCas9-complex, showing force versus number of unzipped base pairs. As baseline, naked DNA unzipping is presented in grey. Insets show zoomed-in view of regions with force peaks with dashed lines illustrating dSaCas9/DNA interaction sites.
Application note | Gene editing