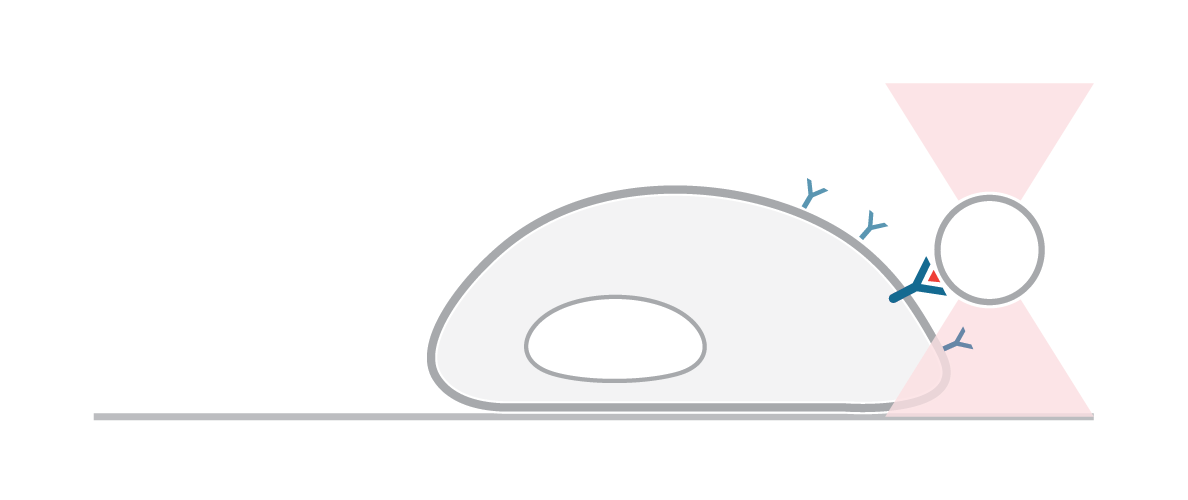
Kinetochore-mediated chromosome segregation
In this experiment, a microtubule was immobilized to a biotinylated coverslip, and Ndc80 complexes, a key protein complex in the kinetochore structure, were attached to streptavidin-coated beads. By moving the bead with the C-Trap, we generated a rupture force between the microtubule and the kinetochore structure.
Researchers from David Barford’s team quantitatively assessed the outer kinetochore’s force resistance of the Dam1 and Ndc80 complexes. By comparing rupture forces of various mutations, they get a better understanding of which protein domains influence the kinetochore’s ability to withstand microtubule forces during cell division.
Cellular tension propagation revisited
Measuring membrane tension is challenging as it requires precise manipulation and measurement of mechanical forces on individual tethers, which are small and difficult to control. With the C-Trap this could be resolved by combining optical tweezers for direct measurement of mechanical forces on individual tethers with optogenetics for local endogenous control of cell protrusion.
By using this combination of techniques, the lab of Bustamante was able to directly measure changes in membrane tension in response to mechanical forces generated by actin-driven protrusions. This allowed them to demonstrate that these protrusions generate rapid long-range membrane tension propagation in cells, which provides new insights into how cells respond to external stimuli and adapt to their environment.
Left: A dual-tether pulling assay to simultaneously monitor membrane tension on the far end (left, trap 1 at 180°) and on the side of the cell (top, trap 2 at 90°) during light-activated protrusion.
Right: Representative time traces of dual trap forces over successive cycles of light-activated protrusion show coinciding tension increases on both membrane tethers adjacent to (trap 2) and at the opposite cell surface from (trap 1) protrusion; light: 90 s on (shaded area), 180 s off.
Figure from De Belly et al. (2023), Cell
The first author of the publication explains how she used C-Trap for the research
Receptor responses to mechanical stimuli
In this experiment, we used the C-Trap to understand the properties involved in the mechanical activation of transmembrane receptors. While applying a controlled mechanical stimulus, we monitored the activation of intracellular responses and signals in real time.
We guided a microscopic bead to the cell surface of a human HEK293 cell expressing fluorescently labeled biotinylated receptors (blue signal) and cytosolic marker (red signal).
Figure 1 shows how moving the bead away from the cell surface with forces above 300 piconewtons caused deformation of the cell membrane and accumulation of receptors at the site of manipulation. As we proceeded, minimal applied forces on the cell membrane were enough to pull out a thick membrane tube.
These observations suggest a correlation between cell membrane deformation and local accumulation of receptor protein at the site of manipulation
We moved the bead in proximity to the D. discoideum cell, ectopically expressing GFP-Myosin 7 (blue signal) and actin filament marker RFP-LifeAct. The cell moved in the direction of the trapped bead, extending multiple protrusions towards it upon sensing the proximity of the object.
We observed a characteristic signal every time the cell engulfed the bead (Figure 2). Interestingly, we also found an occasional force spike right before bead engulfment. Since we observed the formation and retraction of one of the formed filopodia touching the bead, we suggest that this peak possibly is caused by the interaction between the cell protrusion and the bead. Thus, the maximum value shown on the graph (around 20 pN) corresponds to the force that a single protrusion exerted over the foreign object.
Cellular droplet fusion and small components in a multicellular organism
The C-Trap can also manipulate small components inside of living multicellular organisms. We used the optical tweezers to manipulate intrinsic lipid droplets and study droplet fusion inside of Caenorhabditis elegans (roundworm).
Using brightfield microscopy, we can quickly scan cell samples with a micrometer-precision stage and, on top of that, obtain fine movements when needed using a nanometer-precision positioning system. These features allow us to optically trap droplets in two ways: dragging the droplets around the body, thus exposing them to each other, or keeping them at the same position and letting the stage bring other droplets into the proximity of the trapped molecule.
The approach allowed us to follow the small components in vivo and in real time and assess forces associated with the fusion process (Figure 3).